2.1. What is mass extinction?
Mass extinction is the term used by geologists to describe episodes in Earth history when large numbers of fossil species disappear from the rock record during a short period of geological time. Mass extinctions are distinct from the steady rate of extinction which is observed to have occurred continuously through the geological record; that is often called background extinction >>.
|
Mass extinctions therefore indicate something drastic and rapid happened to the Earth surface to cause such a large disruption in the environment that huge numbers of creatures have not been able to adapt, and have died out.
|
2.2. How many mass extinctions?
This is a debatable question, and it depends on how the species are grouped together for analysis. Note that because there is such a large number of fossil species, and they occur over such a long period of time, that it is necessary to group data together to identify patterns. >>
|
The famous work of Jack Sepkoski, using a grouping called family level in fossil organisms, identified 5 major mass extinction events in the period of Earth history where shelly fossils can be easily found. Thus they are called the Big Five and are named as:
|
1. Late Ordovician event, ca. 440 million years ago
2. Late Devonian event, ca. 350 million years ago
3. End-Permian event, ca 250 million years ago; the most severe event: approximately 90% of all marine species died out, and a large number of terrestrial species too.
4. Late Triassic event, ca, 200 million years ago.
5. End-Cretaceous event, otherwise called the K/T (meaning Cretaceous/Tertiary boundary, i.e. the boundary between the Cretaceous and Tertiary Periods of geological time), ca. 65 million years ago (this is the one that killed the dinosaurs).
Geologists are always interested in the date when something happened, and the study of geological time is itself a huge area of interest. Figure 1 below is a simple diagram showing the names of major episodes of geological history, and the position of the Big Five extinctions are shown.
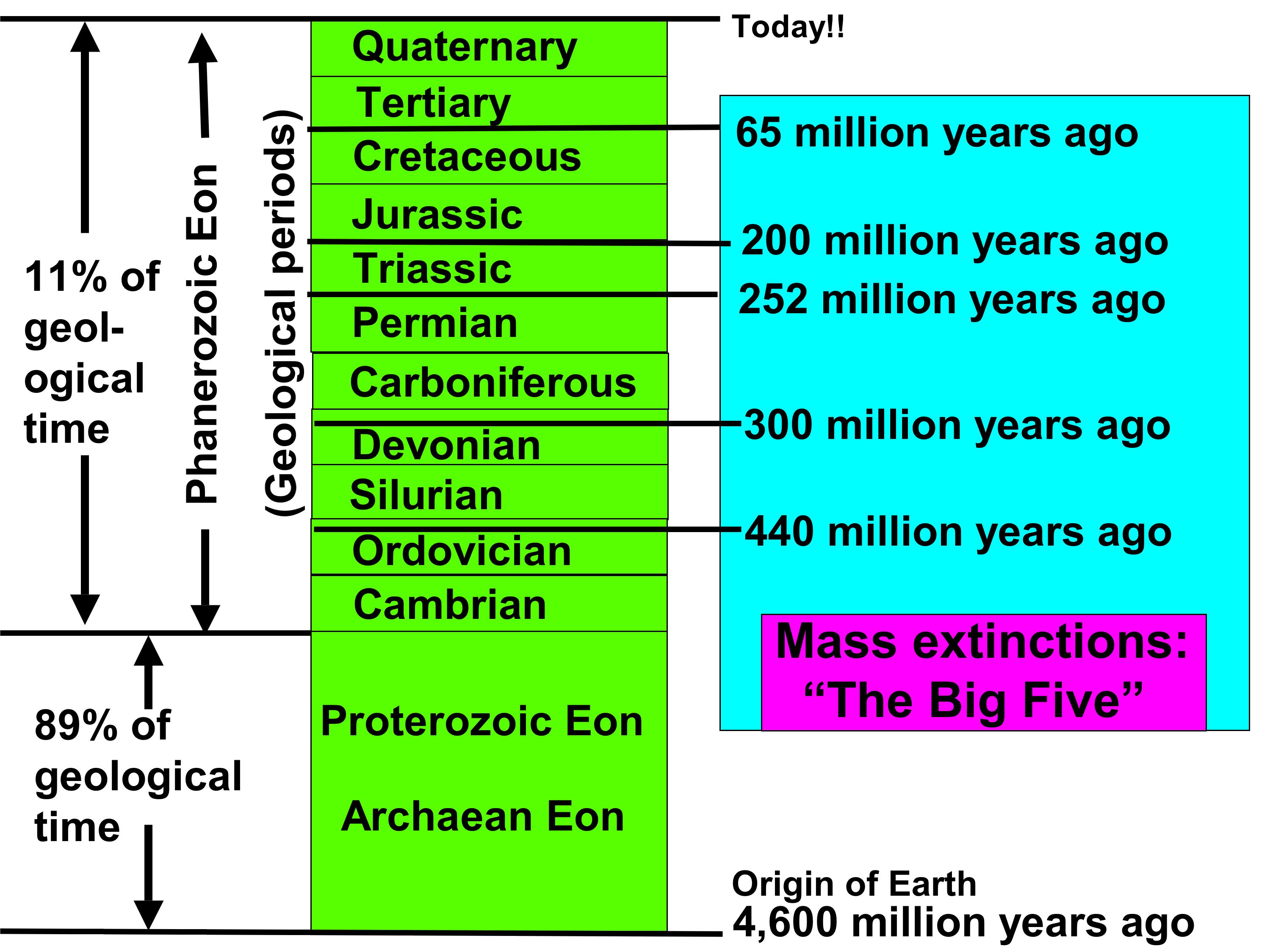
Figure 1: a simple geological time chart showing the names of the geological time divisions, and the times of each of the Big Five mass extinctions in the blue box on the right side. Major time periods are called Eons, and there are three shown in the diagram: Archean, Proterozoic and Phanerozoic Eons. The most recent (Phanerozoic Eon) is composed of a number of subdivisions, called Periods, starting with Cambrian, and ending at the present day with Quaternary. Read the text for details of the mass extinctions.
Some geologists are now thinking that there may be more than 5, depending on how much and how rapidly the disappearance of species is viewed. But the Big Five really are the biggest extinctions, and give us a convenient framework for thinking about the concepts and processes of mass extinction.
2.3. What caused mass extinctions?
All the big five are different, in terms of how severe, what organisms died, their geographic patterns, and what events took place on the Earth surface at the time the extinction happened. To give you a broad idea, the most popular causes of the extinctions are:
1. Late Ordovician event: a rapid glaciation that lasted about 1 million years during a period of time when the Earth was generally very warm. In contrast the modern climate is relatively cool, and the ice sheets we are so familiar with in the north and south polar regions formed about 15 million years ago. Thus the shock of a rapid cooling may have been too much for the biosphere to cope with, and this led to mass extinction.
2. Late Devonian event: This was a time when shallow water anoxia has been recognized in the world oceans, and it is strongly suspected that some oceanographic catastrophe led to anoxic waters. Anoxia means that no free oxygen is present in the water; instead the waters were rich in hydrogen sulphide, as in the deeper waters of the modern Black Sea. Anoxic waters are toxic to all oxygen-dependent organisms, so that only a limited range of creatures can live in it, and these do NOT include the shelly and bony fossil animals we are so familiar with. However, other evidence suggests that there was an overall climatic cooling during this time, and the possibility exists that multiple changes led to this extinction.
3. End-Permian event: Evidence of anoxia is also recorded through much of the world oceans, but there was also a giant volcanic eruption in Siberia, producing lava up to 1 km thick across an area of half of Russia. It is this coincidence of globally-reaching disasters that might have led to such a severe extinction.
4. Late Triassic event: This coincides with a substantial volcanic event, which could be the cause.
5. End-Cretaceous (K/T) event: this is famously attributed to a meteorite impact, which is accepted by almost all researchers, with substantial evidence. A giant meteorite hitting the Earth, in the present location of the Yucatan Peninsula of central America, is interpreted to have caused such a cataclysmic disruption to the biosphere that it caused the dinosaurs to go extinct on land, along with lots of other creatures, including the famous ammonite organisms in the sea; ammonites are familiar to anybody who has visited the area of Lyme Regis on the southern UK coastline, and are iconic in the perception of fossils in the UK.
With the exception of the K/T event, which is generally accepted to have been caused by a meteorite impact, it is likely that mass extinctions were caused by coincidence of different types of environmental catastrophes generated within on the Earth, without external influence, which the biosphere could not adapt to. Any one event on its own may not have been so bad, but the combination of events that have different underlying causes may be the ultimate cause of mass extinctions. Thus it is an interesting truth that the biggest event in history, the end-Permian extinction, is not associated with evidence of a meteorite impact. >>
|
Therefore the wealth of evidence indicates that the end-Permian event was a home-grown extinction. It has been suggested by some researchers that a major reason why so many fossil animals disappear at the end-Permian extinction event is that their physiology was unable to cope with the environmental changes associated with a significant rise in carbon dioxide in the atmosphere and oceans, related to the Siberian volcanic eruption. In particular, data compilations have been used to interpret that those animals with heavily calcified shells were most affected. The disappearance of these animals may have left an open ecological space in the oceans, which was taken up by microbial structures that are the subject of my research, and illustrated in Section 3 of this document.
|
3) MICROBIAL STRUCTURES (MICROBIALITES)
3.1. What are microbial structures (microbialites)?
Sedimentary rocks are well-known throughout geological time, and may be considered to be composed of two types of material:
a) inorganic particles and precipitates, on the sea floor, lakes, rivers, deserts. Familiar material such as silica (silicon dioxide) forms sand (which becomes sandstone when lithified); clay forms shale and mudstone; calcite (calcium carbonate) forms limestone; sodium chloride forms salt, calcium sulphate forms gypsum; and there are many other inorganic materials. >>
|
b) organic components include organic carbon (e.g. plant matter that makes coal), inorganic carbon (calcium carbonate that forms shells of animals, some plants and some microbes); silica (which forms parts of sponges, and also extremely important in planktonic oceanic plants called diatoms which make up about half the photosynthesising phytoplankton of the world oceans). There is also mineral matter that has been precipitated by action of microbes living in the environment, normally silica and calcium carbonate, but others occur, for example iron oxides and iron sulphides.
|
Microbes are preserved in the fossil record in two major forms, visually observable in rock specimens and microscope sections:
a) Calcimicrobes: some microbes precipitate mineral matter and form solid structures that are effectively shells; they may also be called skeletonised microbes;
b) sediment microbialites: other microbes merely influence the sediment and cause it to clump, or form distinct layers; in these cases there is normally little or no visible microbial matter preserved, but the sediment contains structures which can reasonably be interpreted as having formed by the action of microbes. Such structures do not contain any microbial shells, so they are not called calcimicrobes; instead other terms are used, and the two most common types are stromatolites and thrombolites.
Recent work on organic chemicals preserved in sedimentary rocks has led to interpretations that biomarkers for microbes can also be found; these require chemical analysis and cannot be visually observed in rock specimens and microscope sections.
It is possible for a microbial structure to contain a mixture of calcimicrobes and/or one or more of the sediment microbialite fabrics, and so they can develop into quite complex structures. To make matters worse, there are some stromatolites that seem to be formed entirely out of calcium carbonate crystals (called cement) with no proof of any organic action. Because of such complexities, there have been difficulties with naming them, but the above two-fold division into calcimicrobes and sediment microbialites works well for most cases.
3.2. Why are microbialites important in the world?
Microbialites occur throughout the geological record, and are common on Earth today. It is well-recognised that microbial processes are crucial for the operation of ecosystems, and even the physiology of individual organisms; for example the human digestive system depends on microbial creatures for the success of digestion of the food you eat. >>
|
In the geological world, microbialites play a significant role in the formation of sedimentary rocks in many places; they may be made of a wide variety of mineral material, but the two most common materials are silica (silicon dioxide) and calcium carbonate (which is what limestone is made of). In mass extinctions, it is the calcium carbonate forms which are found.
|
Illustrations below show fossil types that might be more familiar to you (Figure 2), and then some microbialites (Figure 3), so you can see the differences between them.

Figure 2: Photographs of familiar fossils found in normal Earth surface environments, not related to extinctions. Thus these are organisms which may be found as part of normal highly diverse biological communities, indicating a complex ecosystem that is normal on Earth. After extinction events, such communities are destroyed and tend to be replaced by simple communities dominated by lower life forms such as microbialites illustrated below in Figure 3.

Figure 3: Photographs of unfamiliar fossils found in Earth surface environments which are commonly stressed, and are the kinds of organisms commonly found as fossils in the rock record immediately after extinction events. These three photographs show stromatolites, from modern and ancient examples. Figure 3A (above) shows stromatolites from the famous Cotham Marble from the Bristol area in west England. Figures 3B and 3C are shown below the next text block.
Please note that stromatolites are actually quite common in the Earth surface marine environments today, but tend to dominate in places where the environments are stressed. After mass extinctions, these organisms dominate environments, and the normal marine fossils illustrated in Figure 2 tend to either disappear or occur in much smaller number and are less biologically diverse. >>
|
The distribution of organisms on Earth after extinctions is quite complicated, and the exact ecosystems that develop from place to place are governed by regional variations in the extinction. It is therefore dangerous to overgeneralise about extinctions; for example, after the famous K/T extinction, mentioned in Section 2 of this topic on the previous page, there are no microbialites recorded, yet the extinction was highly significant on the Earth surface.
|
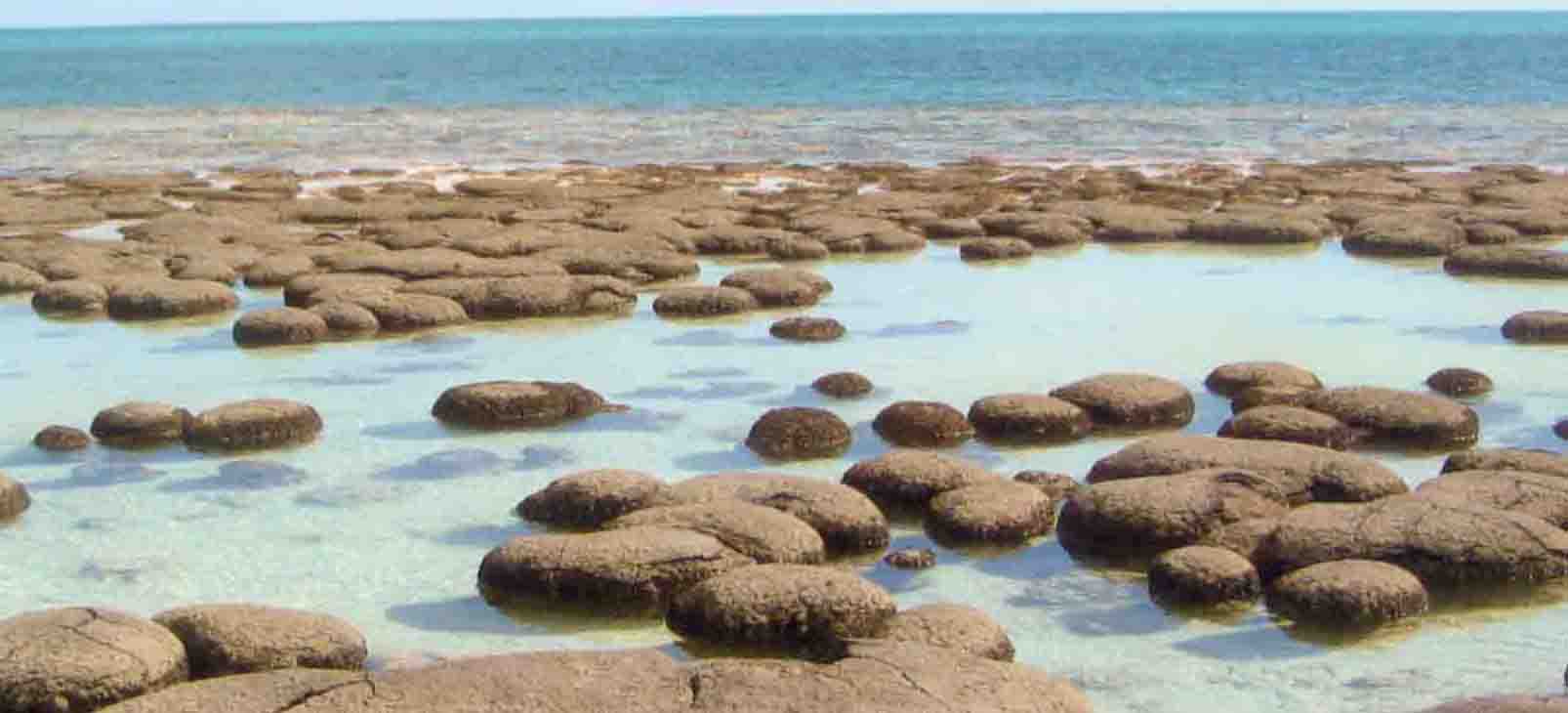
Figure 3B: Photograph of modern-day Shark Bay in Western Australia, showing living stromatolites in a highly saline bay, where they survive well in this stressed environment. This location is famous to geologists because it is used as a modern representation of what some ancient shallow marine environments are thought to have looked like; in particular it fits with the views of many people of the shallow sea directly after the end-Permian mass extinction.

Figure 3C: Photograph of a microscope view of a stromatolite from a geologically-recent coral reef in the Gulf of Corinth, Greece, at a locality called Mavra Litharia. This reef is actually rich in corals and shells and is nothing to do with mass extinction. Therefore the presence of a stromatolite in its structure is simply a reflection of the diverse biological community making the reef, in a non-extinction setting.
Remember earlier, the terms STROMATOLITE and THROMBOLITE were mentioned? Figure 4 below shows that stromatolite and thrombolite are two types of microbialite.

Figure 4: Simple graphic showing that stromatolite and thrombolite are two types of microbialite.
Figures 5 and 6 below show the key features of stromatolite and thrombolite; these two words are essentially field-based terms, and emphasise the layered construction of stromatolites (the Greek word stroma means a bed or mattress) and thrombolites (Greek thrombos means blood clot).

Figure 5: Photograph of a vertical section of a stromatolite, from the end-Permian mass extinction beds in southern Turkey.
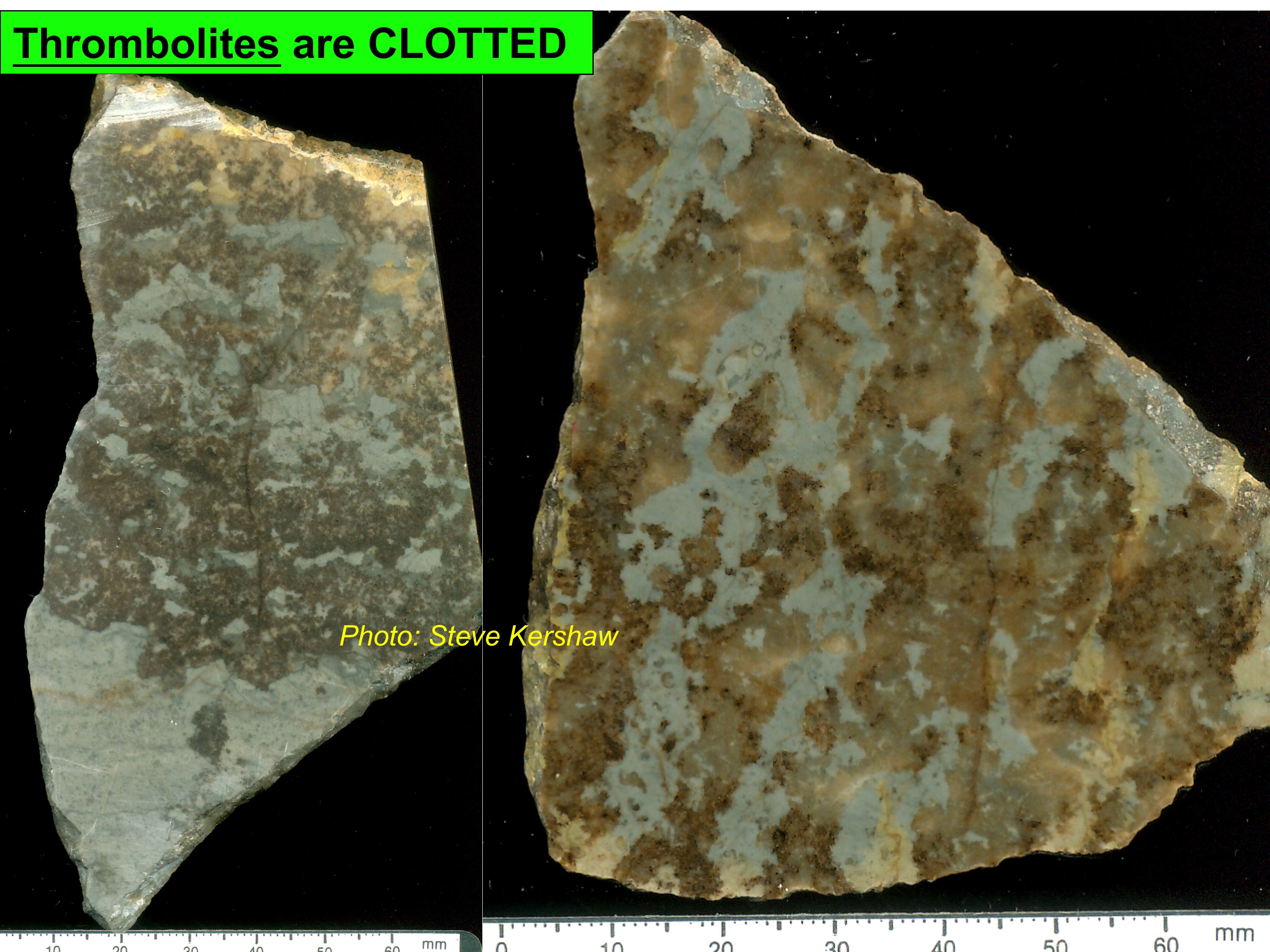
Figure 6: Photograph of a vertical section of two polished rock pieces of thrombolite, from the end-Permian mass extinction beds in southern China.
The photos in Figures 5 and 6 above show microbialites made of layered and clotted sedimentary material, but Figure 7 below shows a microbialite made of constructed tubes of a cyanobacterium called Rivularia, which is therefore a CALCIMICROBE.

Figure 7: Photograph of a vertical microscope section of Rivularia, a calcimicrobe microbialite from the Pleistocene age rocks in Perachora Peninsula, north of Corinth in the Gulf of Corinth, Greece. This fossil is constructed of tubes and is therefore a skeletal microbialite, rather than the sedimentary microbialites of Figures 5 and 6.
4) MICROBIALITES AFTER THE END-PERMIAN MASS EXTINCTION
4.1. Why are microbialites important in mass extinctions?
Actually microbialites are important in only some mass extinctions, and the reasons are not understood. There is some evidence of microbialites after the Late Ordovician and Late Devonian extinctions, none after the Late Triassic and end-Cretaceous extinctions, but they are very abundant after the end-Permian extinction. There are scores of studies that try to unravel the reasons for these differences, and there is no clear answer. The ideas fall into two general areas:
1. Microbialites form in conditions of high levels of available material for making calcium carbonate structures; this is called 'saturation' of the ocean water with respect to calcium carbonate. >>
|
2. Microbialites occupy the ecological space left empty by the extinction of shelly and bony creatures, and the rather catchy phrase DISASTER BIOTAS has taken hold in the literature.
Thus, those mass extinctions that relate to these two concepts tend to have microbialites formed immediately after the extinction. However, the fundamental reason(s) why microbialites form, or do not form, after mass extinctions is/are still not understood.
|
4.2. Places to study microbialites; strange world of the end-Permian Period
The end-Permian mass extinction affected the whole world, and microbialites occur in a large variety of sites. However, continents of the Earth are in motion, and 250 million years ago, the configuration of continents was somewhat different from today, and the world map can be divided into three major features:
Pangaea: the supercontinent containing nearly all the continents, except for a few in the eastern region. Panthalassa: the major ocean that occupied most of the rest of the Earth surface. This might also be called Oceanus, but the name Panthalassa has stuck.>>
|
Tethys: Tethys was the wife of Oceanus in Greek mythology, so the sea that exists in the eastern part of Pangaea is called Tethys. Note that actually there are two Tethys Seas: a northern part (old Tethys, called PalaeoTethys), and a southern part (young Tethys, called NeoTethys); they are separated by a string of continents.
The map below in Figure 8 shows the locations of Pangaea, Panthalassa and Tethys, and also the main area of occurrence of microbialites after the end-Permian extinction, which is in Tethys.
|
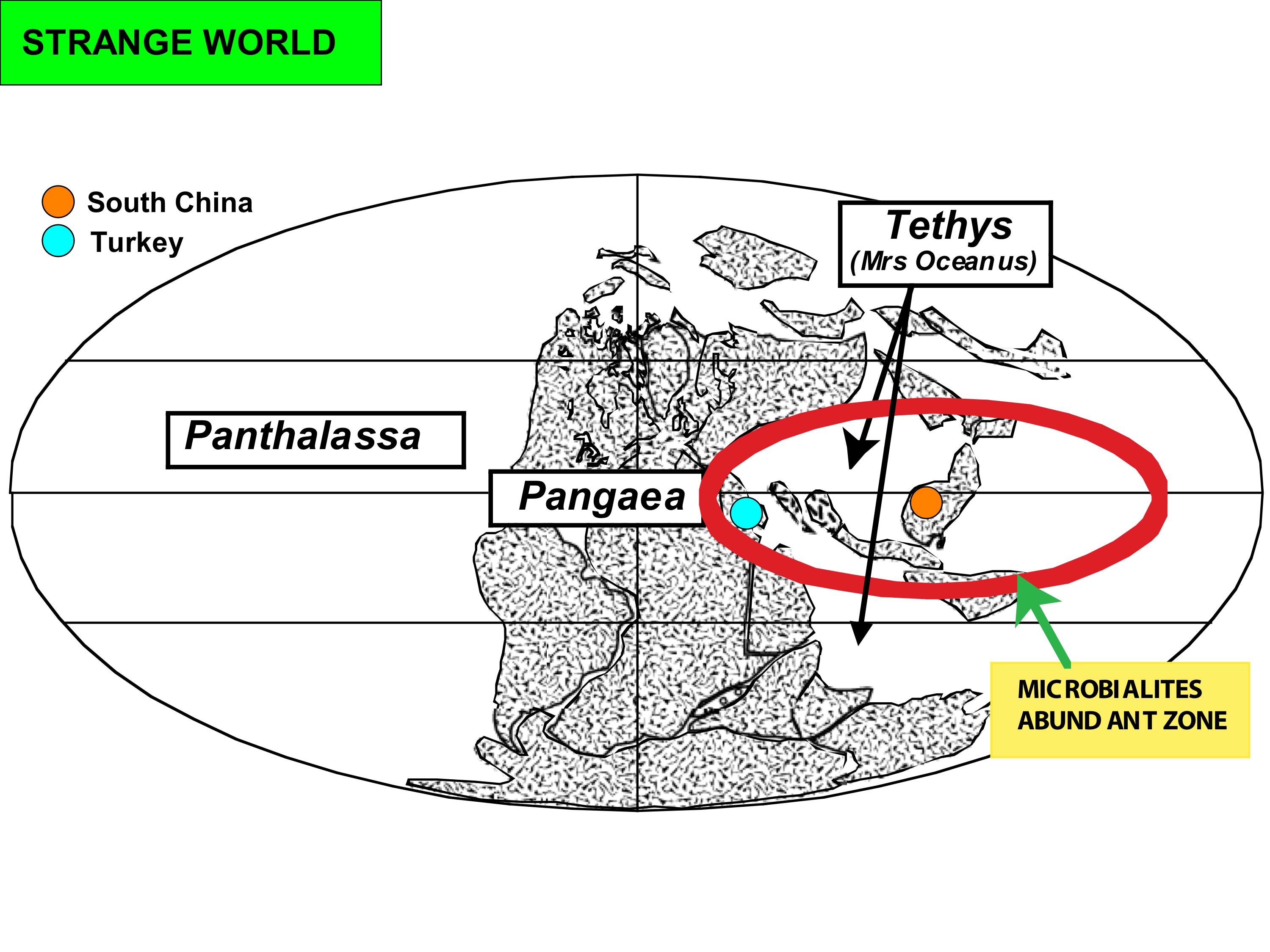
Figure 8: Map of the world during the time of the end-Permian mass extinction; this is called a PALAEOGEOGRAPHIC MAP; notice how the continents are in different places from the present day, but you can recognise the outline of the major continents. The examples shown in figures below are taken from places in south China and Turkey, the palaeogeographic positions of which are highlighted on this map.
4.3. Before the end-Permian mass extinction
To give you an idea of the rich diversity of life before the end-Permian mass extinction, here are some photos of fossils found in the reefs directly before the extinction took place.
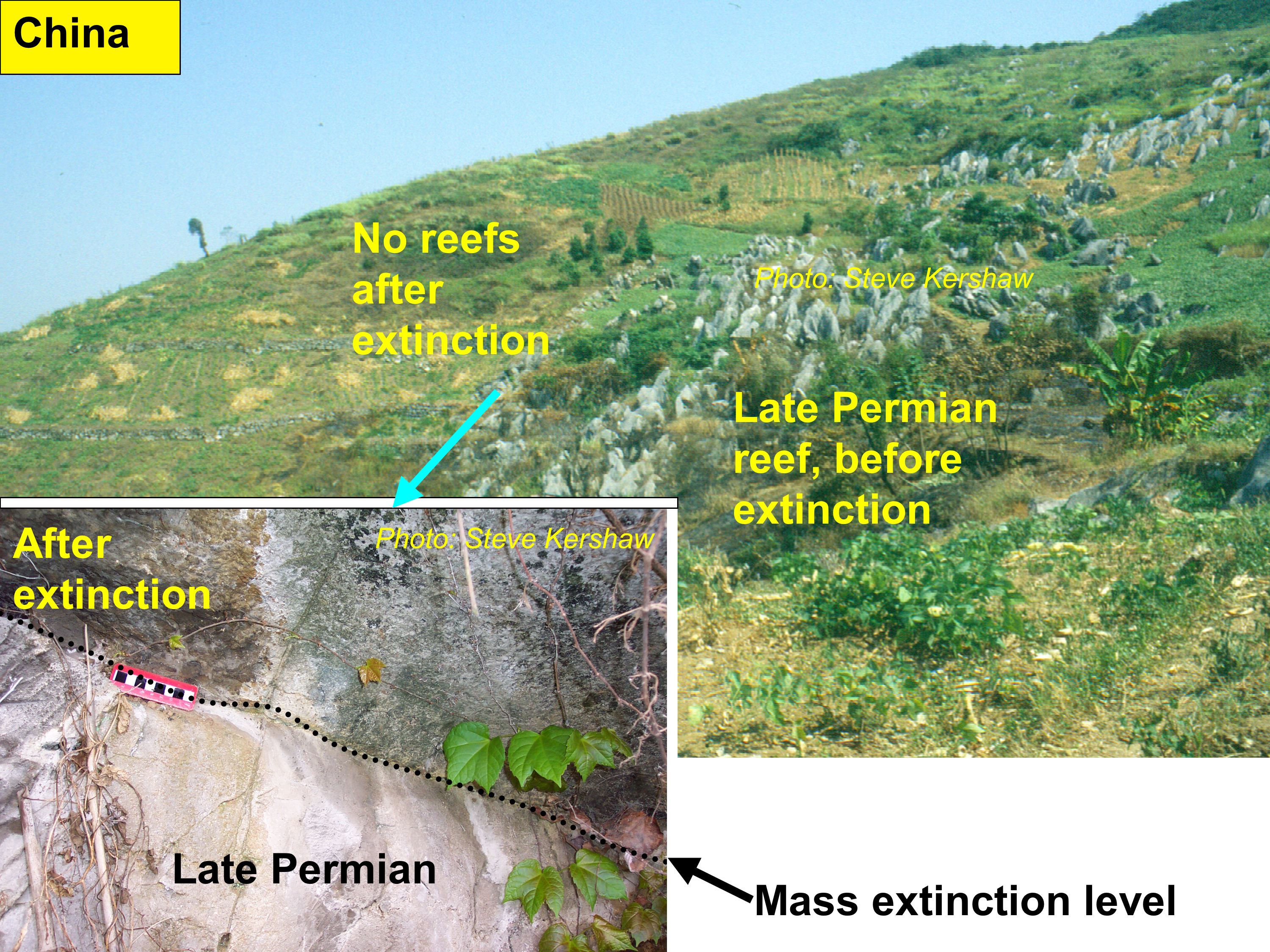
Figure 9: MAIN PHOTO: a famous site in Chongqing Special Administrative Region (SAR) in central China, showing a hillside that includes rocks crossing the end-Permian extinction event and the Permian-Triassic boundary. The grey rocks in the middle of the picture, protruding from the ground, are a latest Permian fossil reef. The reef dies out just below the extinction event, and it is noteworthy that the central China reefs are the youngest reefs of that age in the world, disappearing just before the extinction event; at that time the world sea level had fallen to its lowest level of the entire geological record, which may the prime reason for the loss of reefs. INSET PHOTO: a close-up photo from another site that shows the contact between the latest Permian rocks and the rocks immediately after extinction: those upper rocks are microbialites.
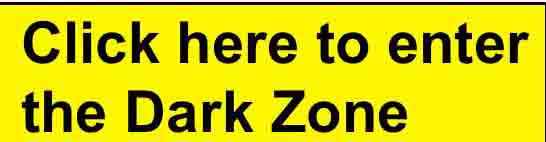
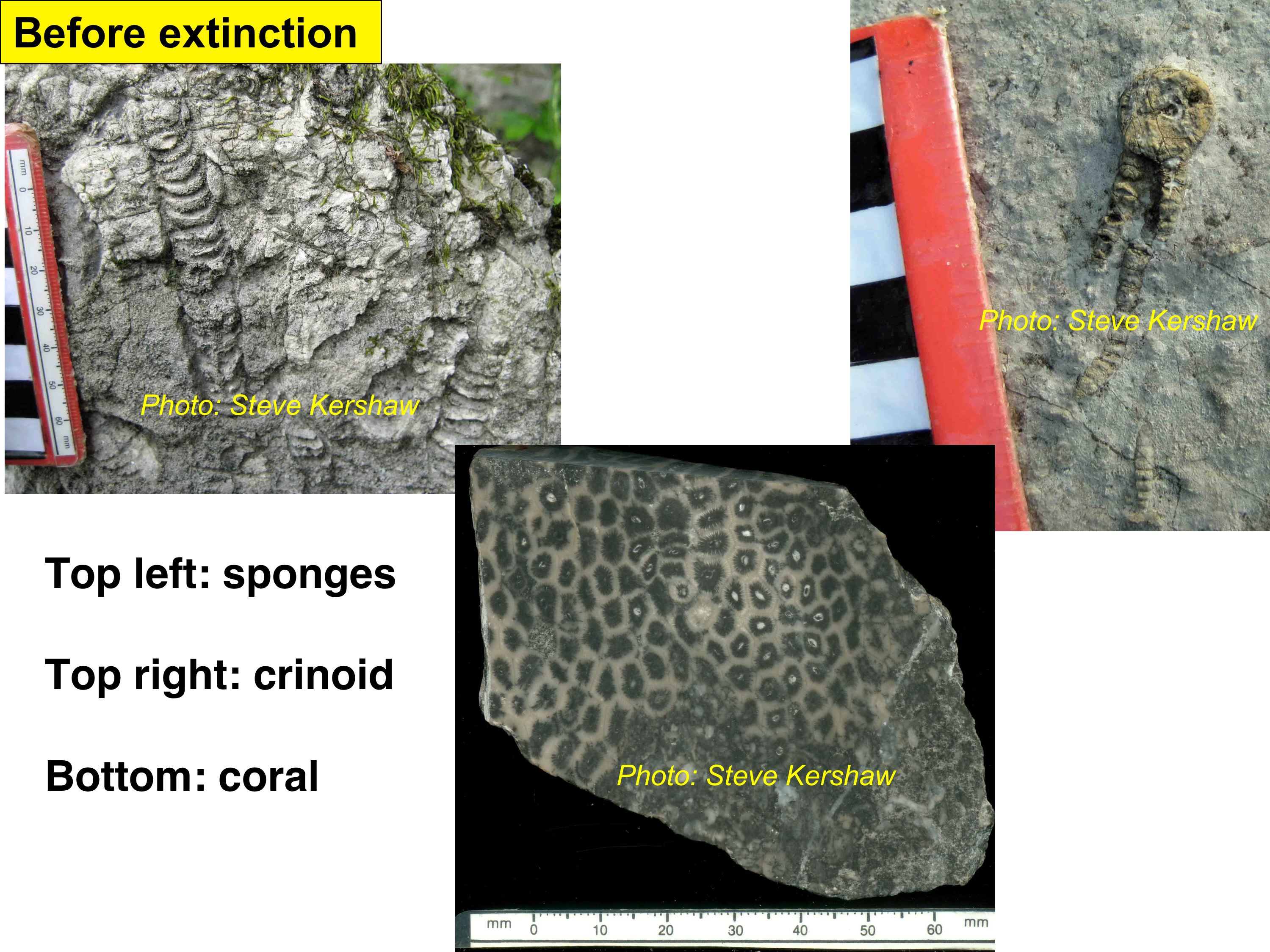
Figure 10: Three photographs of typical fossils present in the latest Permian rocks before the extinction.
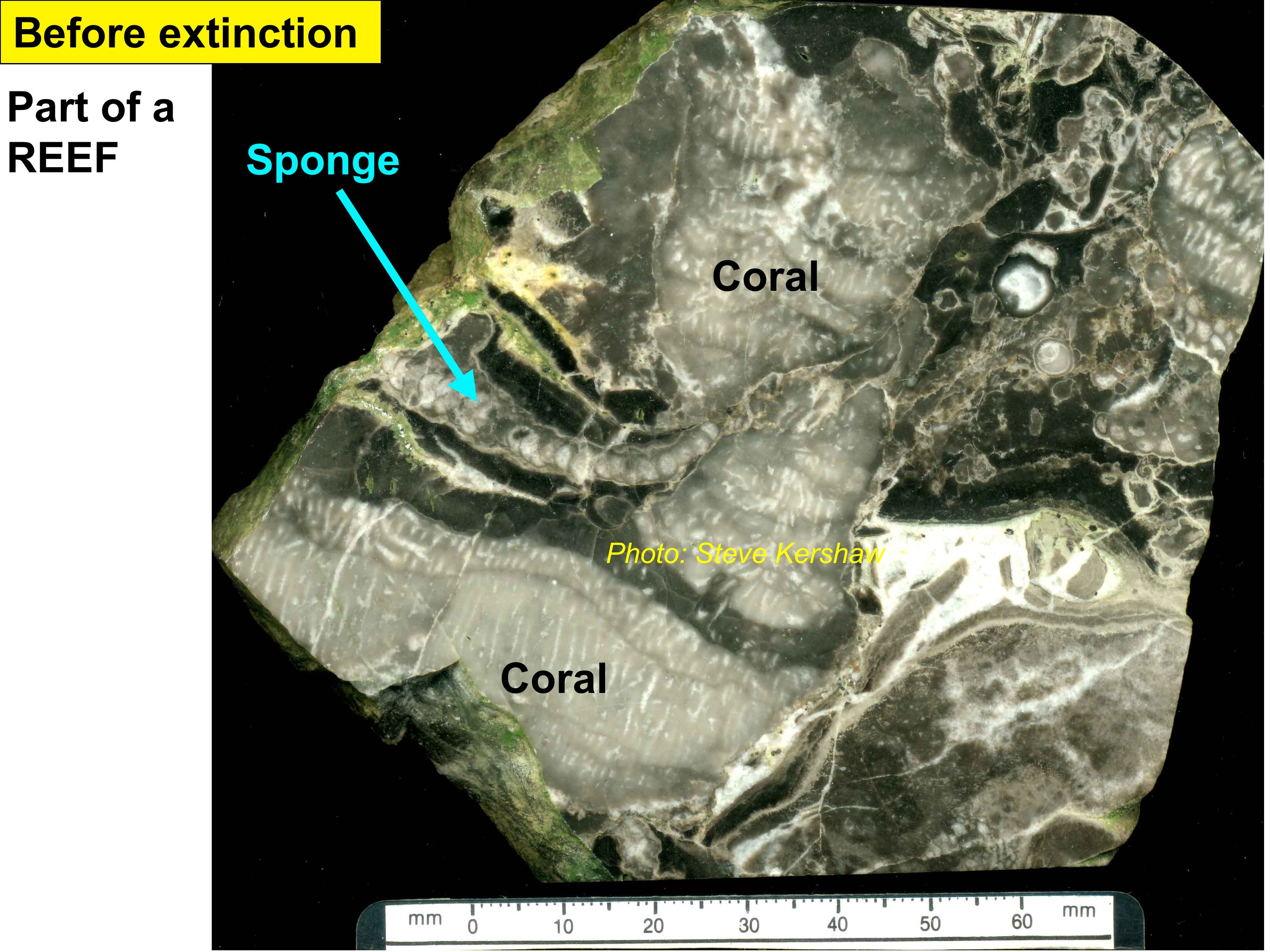
Figure 11: Polished vertical section of a piece of latest Permian reef rock, comprising corals and sponges.
4.4. Microbialites after the end-Permian extinction
After the extinction, the diversity of pre-extinction fossils disappears, and is replaced by a microbial world of stromatolites, thrombolites and calcimicrobes. The illustrations below show the range of structures, using Turkey and South China as examples.

Figure 12: polished blocks of vertical sections of microbialites from southern Turkey. The difference between the clotted structure of thrombolite (top) and layered structure of stromatolite (bottom) is clearly demonstrated in these photos.
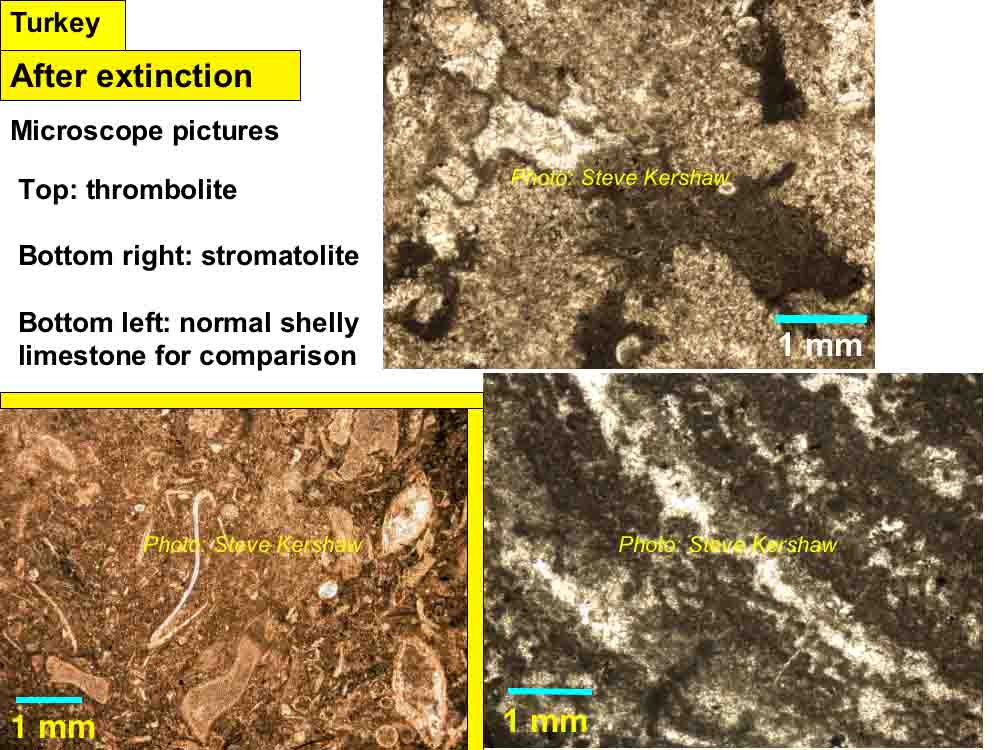
Figure 13, top and bottom right: microscope views of vertical sections of thrombolite and stromatolites, from the samples in Figure 12. The bottom left photo is a microscope view of a normal (non-extinction) limestone containing shelly fossils, for comparison with the post-extinction microbialites.

Figure 14: Layered microbialites after the end-Permian mass extinction in south China. Notice the flat layers at the bottom and the more domed layers higher up.

Figure 15: close-up of the upper part of the microbialite in Figure 14 (from a different location but the structure is the same). Note the vertically-orientated branches of microbialite (grey colour), and lighter coloured sediment deposited in between the branches.

Figure 16: 3-dimensional diagrammatic reconstruction of the vertically-branched microbialite shown in Figures 14 and 15. In close-up on the right, the surface of the branches is shown as covered with rounded lumps; these are based on real structures that are not visible in Figures 14 and 15 because these are cut sections and the surface cannot be seen. However, Figure 17 shows the nature of these lumps.
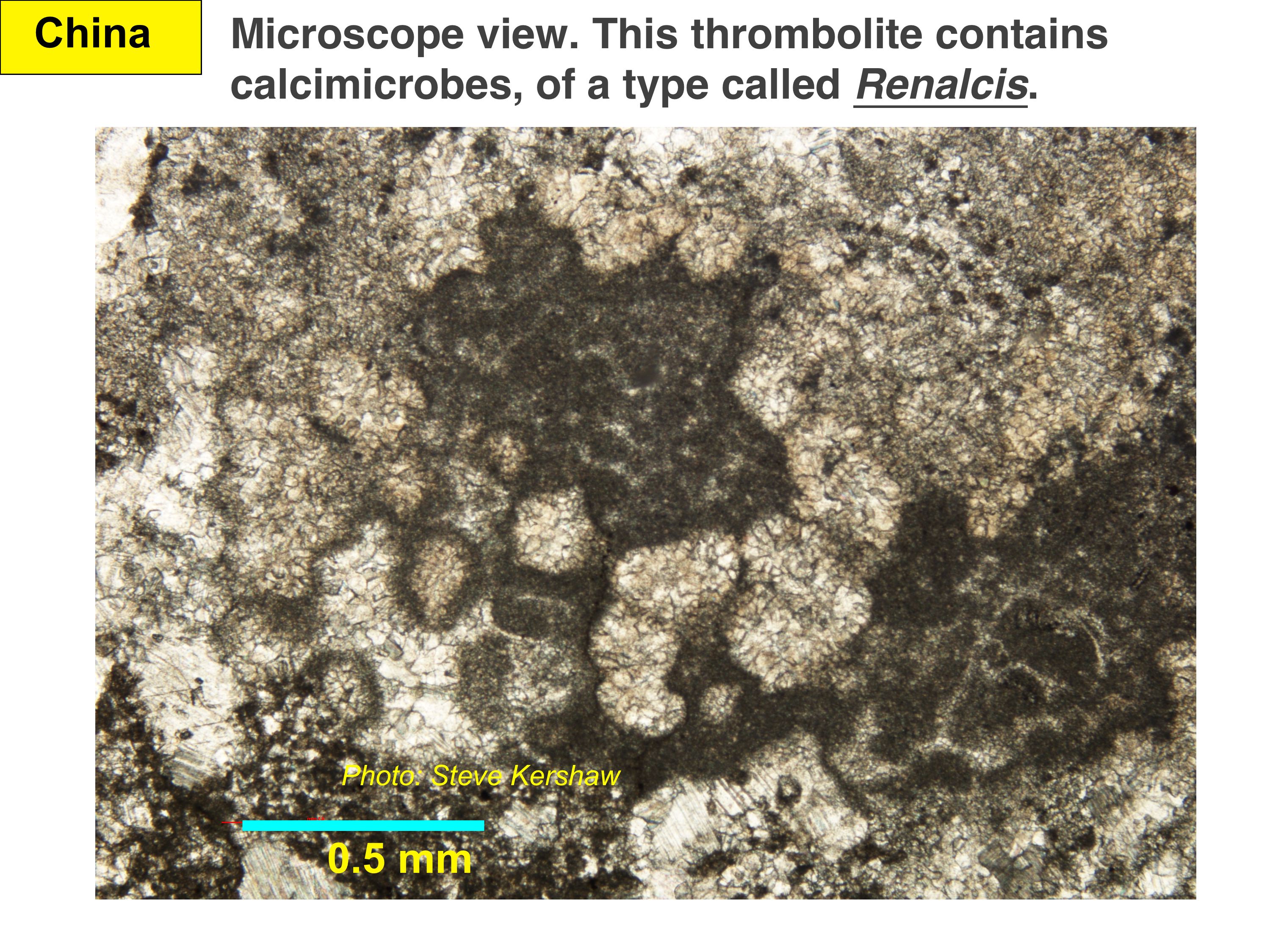
Figure 17: microscope section of the microbialite of Figures 14 - 16, showing the lumps as near-circular structures. These are calcimicrobes (remember this term from earlier on this page?). The calcimicrobe is called Renalcis and interpreted as cyanobacteria - i.e. photosynthesising bacteria. The tiny dark blobs in between the lumps are sediment deposited between the Renalcis spheroids.
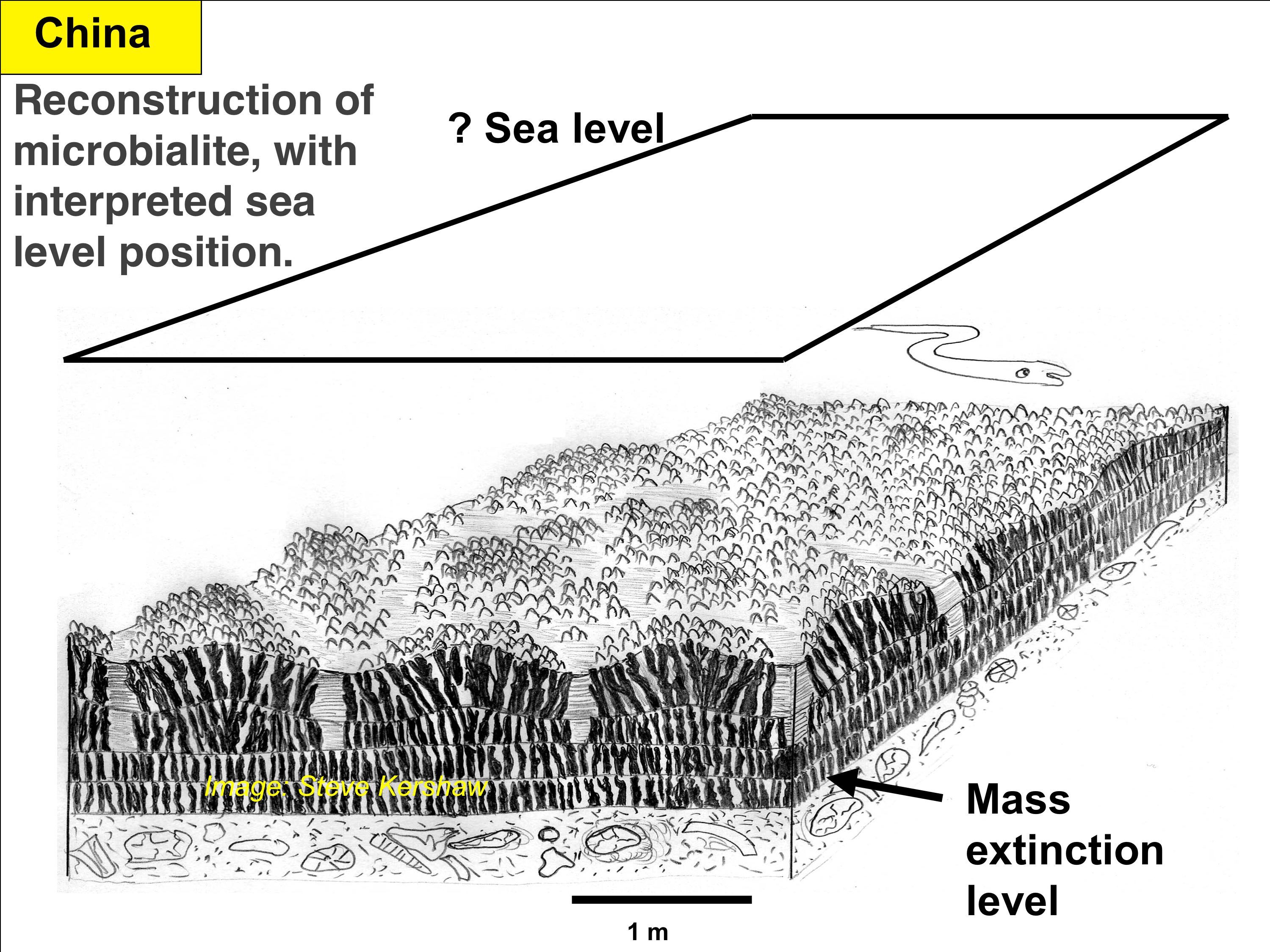
Figure 18: Reconstruction diagram in three dimensions of the microbialites from China shown Figures 14-17. The estimated position of sea level is shown.

Figure 19: Reconstruction diagram in three dimensions of the microbialites from Turkey shown in Figures 12 and 13. Notice how this is different from Figure 18, because they occur in different parts of the ancient world (compare the locations of Turkey and south China in Figure 8).
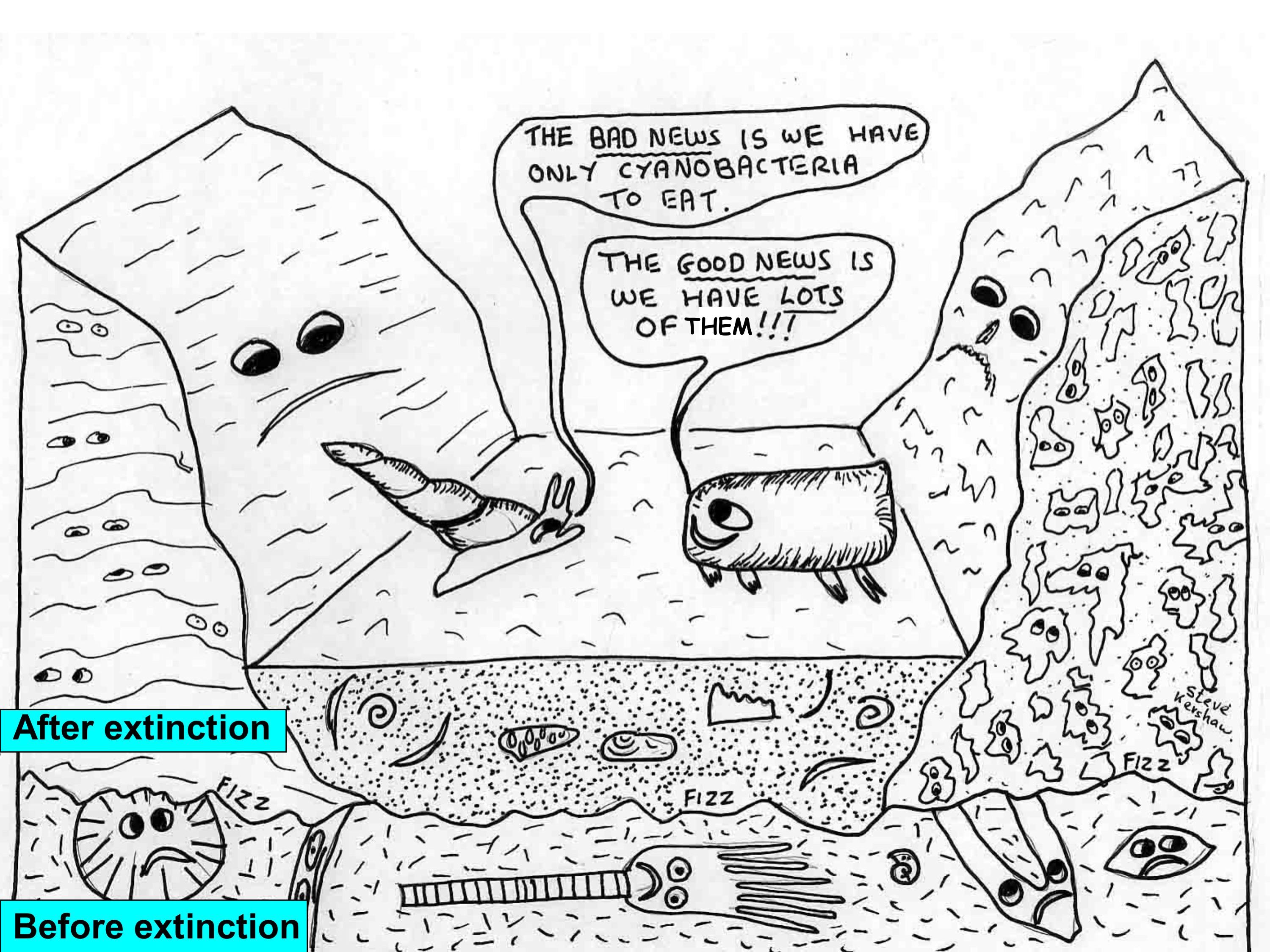
Figure 20: This cartoon joke has a serious point: the extinction did not destroy the whole ecosystem. Yes it wiped out lots of organisms, but very quickly afterwards the ecosystems were rapidly reconstructed, although consisted of communities that were dominated by bacterial organisms. Thus the bacteria form the food supply for small shelly organisms, represented in this cartoon by small snails and tiny crustaceans called ostracodes.
5) WHAT CAUSED THE MASS EXTINCTION, AND WHAT ARE THE IMPLICATIONS FOR CURRENT CLIMATE CHANGE CONCERNS?
5.1. Volcanic eruption
There is ample evidence of a major volcanic eruption around the time of the mass extinction; it is in Siberia, producing a sequence of lavas called the Siberian Traps, covering an area half the size of Russia up to 1 km thick. This is the largest mass extinction in the geological record and is presumed to have injected large amounts of carbon dioxide into the atmosphere, to cause a >>
|
large global warming event, that might have destabilised the Earth surface environments and contributed to the extinction. Furthermore, the eruption is known to have occurred through a series of rocks rich in chlorine (salt) and sulphur (gypsum), and these may have released substantial quantities of toxic gases.
|

Figure 21: Simple diagram showing some major environmentally-related events on Earth surface related to the mass extinction. See the text below for details. By the way, the volcano photo is from Lanzarote, of Tertiary age, nothing to do with the end-Permian mass extinction!
In Figure 21 there is an apparently obvious question; of course there is no volcanic super-eruption taking place today, but it has been estimated that the amount of carbon dioxide being emitted by human activity exceeds all the output by volcanoes around the world. >>
|
So, it might be argued that the human production of carbon dioxide into the atmosphere-ocean system has an equivalence to a large volcanic eruption; something to think about.
|
5.2. Ocean oxygenation problems
The modern ocean is oxygenated by circulation of global ocean waters, but in the end-Permian and Early Triassic times, there is evidence of reduced levels of oxygen in the deep ocean waters. The ocean circulation system was likely different from modern times, so that the ocean was less vigorously circulating. It has been suggested that the mass extinction was in part caused by disruption of the oceans, and upwelling of low-oxygen waters into the shallow ocean, probably also carrying hydrogen sulphide from the deep. There is good reason to suppose that such toxic gases as hydrogen sulphide can form in the deep ocean, because this is what happens in the modern Black Sea. If upwelling did take place during the end-Permian event, then the result should have been poisoning of the shallow waters and contributing to the extinction.
Whether or not the microbialites were able to survive in low oxygen conditions is not known. The issue is controversial, with evidence both in favour and against this idea. More work will hopefully resolve the issue. >>
|
Nevertheless, the issue of reduced oxygenation of ocean waters is a problem we face today. There are several places in the world oceans where reduced oxygen levels are recorded; for example in the Baltic Sea, and in the northern Pacific and northern Indian Oceans. However, the reason for this is not upwelling of low oxygen water but is more mundane: human agriculture produces a large amount of excess nitrate used for fertilisers, that simply wash off the fields into rivers and make its way to the sea. In the oceans, nitrate stimulates the growth of phytoplankton leading to plankton blooms, which strip oxygen out of the water and reduce its levels, giving problems for the rest of the food chain.
|
Figure 22 below shows an interpretation of upwelling of deep ocean anoxic water to stimulate microbialite growth at the end-Permian mass extinction.

Figure 22: Reconstruction diagram of possible scenario of upwelling of deep low-oxygen oceanic water to the shallow waters; this is one explanation of the cause of extinction, for which there is much evidence in geochemistry of these ancient sediments.
5.3. Ocean acidification
In the end-Permian extinction, sulphur oxides from volcanic eruptions may have dissolved in the ocean waters, and, together with the increased levels of carbon dioxide, are interpreted by some researchers to have caused ocean acidification. There is a lot of current research on modern ocean acidification and it is of grave concern for the oceans; coral reef growth is diminished and there are effects on fishstocks. Thus we have an issue of food security in modern times. The UK government has a document on ocean acidification that you can download from the government website. The current situation is that the acidity of the oceans has increased by 0.1 units of pH. This does not seem much, but it already has measurable negative effects on the ocean health. >>
|
The big problem for the end-Permian event is proving that ocean occurred in those times. Some researchers suggest that it is possible to identify marine dissolution surfaces in the limestones (Figure 23), but these can also be formed above sea level, in a freshwater setting. Such effects are common in limestones around the world today, and they commonly form a landscape called KARST (Figure 24); there are many fine examples of karst in the British countryside. Therefore, while the idea of ocean acidification is logical and sensible for the end-Permian mass extinction, proving that it actually happened is not so clear.
|

Figure 23: Vertical section of polished sample of limestone showing a complex sequence of change. The lowest area of grey sediment is overlain by an area of sediment with white circular patches (these are fossils called foraminifera). Both these two layers of sediment are latest Permian in age; the upper layer has an irregular contact with an overlying dark grey sediment with black irregular patches: this is the post-extinction microbialite. The boundary between the microbialite and the underlying sediment is an erosion surface, and is a surface which has been interpreted by some geologists as representing sea-floor dissolution of the limestone (carbon dioxide dissolved in seawater can potentially dissolve limestone, as happens on land, due to acid rain; for example some buildings made of limestone show this - a very good example can be seen at the top balcony of the Cathedral of St Paul in east London). However, the problem in the sample in this figure is that it is not possible to distinguish between dissolution on land and dissolution in the sea: they produce the same results. Consequently distinguishing between sea-floor dissolution by ocean acidification, and on-land dissolution by acid rain is not possible in these samples, from the current evidence.

Figure 24: Photographs from south China showing karst landscapes: these are landscapes of limestone that has been subjected to tens of millions of years of acid rain on land, with the result being these beautiful sculpted landscapes. Compare these pictures with Figure 23 and the associated text.
To summarise, although we do not have a parallel between the end-Permian event and modern day environments, we do have some elements of similar processes. >>
|
Most people doubt that modern anthropogenic activity of rising atmospheric carbon dioxide, and the accompanying increase in ocean acid, will cause an extinction on the scale of the end-Permian event, but we will have to wait and see!
|
Click here to return to the GENERAL INTEREST PROJECTS header page
Click here to return to the HOMEPAGE